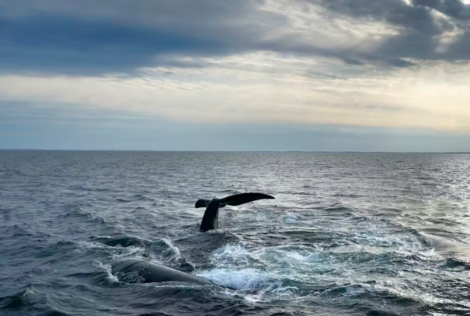

Reducing CO2 emissions is part of the public discourse on addressing climate change, but what are we doing to remove CO2 that’s already been emitted?
The world’s oceans absorb nearly 30 percent of all atmospheric CO2 emissions, acting as nature’s largest sponge. However, this process increases ocean acidity, damaging marine ecosystems. The de Lannoy Lab at McMaster University is focused on technologies to both de-acidify the oceans and sustainably remove CO2 from the atmosphere, safely storing it in the water.
Charles de Lannoy, Associate Professor of Chemical Engineering, emphasizes the urgency of this research. “Technologies for long-term carbon storage are still in their infancy and lack adequate support from governments and the private sector,” he explains.
In collaboration with the Advanced Membranes Lab led by Jay Werber at the University of Toronto and with funding from the Carbon to Sea Initiative, de Lannoy and McMaster post-doctoral fellow Bassel A. Abdelkader are testing a promising carbon removal process. Their technology offers a more cost-effective solution compared to existing methods, potentially paving the way for scalable and affordable CO2 removal.
How it works
de Lannoy and Abdelkader are using an electrochemical approach known as bipolar membrane electrodialysis (BMED), whereby the acidity of seawater is removed thereby enabling increased removal of CO2 from the atmosphere, without the addition of other substances.
The inputs are simple: electricity, seawater and fresh water. The overall system components are more complex and include pumps, membranes electrodes, electrolyte, piping, gaskets, spacers and more.

“The simplicity and modularity inherent to the BMED technology allows a flexible, scalable and potentially cost-effective method of carbon dioxide removal,” says de Lannoy. “BMED shows promise for large-scale implementation, especially when integrated with existing facilities like desalination plants.”
In removing CO2 through BMED, ocean pH will be rebalanced and can increasingly absorb atmospheric CO2.
Abdelkader, who brings expertise in membrane technologies to the de Lannoy lab, has built a lab-pilot model of a BMED system to validate the engineering, minimize operational costs and identify optimal configurations.
The initial results are promising, and we’re now focused on scaling it to improve its potential for real-world application.
Manufacturing membranes
One of the barriers to scaling the project is the exorbitant cost of the membranes used to capture CO2. The paper-thin polymer material is commercially available but at prices that are cost prohibitive. To process vast volumes of water would require huge membrane surface areas, which need to become more affordable before such a large magnitude of OAE is industrially viable.
“A viable membrane option is a critical part of this research,” says Abdelkader. “In addition to cost, commercially available membranes are only lasting three to four months under certain conditions in our testing.”
That’s where Jocelyn Riet, a PhD chemical engineering researcher at the University of Toronto, is lending her expertise.
“Bipolar membranes have charged molecular groups bonded to a polymer scaffold. Charged groups inside the polymer allow ions of the opposite charge to pass through the membrane and block those of the same charge,” Riet explains. “Because of that, when applying an electric field, certain ions will try to pass through the membrane, but they will be stopped, causing water to dissociate into dilute acid and base in the membrane.”
Riet is manufacturing a membrane specifically for McMaster’s BMED testing that lasts longer and hopes to be 10 times less expensive than off-the-shelf materials.
Membranes from U of T will be tested by de Lannoy and Abdelkader to validate their performance; they are hoping for continued success in their testing and eventual buy-in from industry and government partners.
There’s a way, but is there a will?
“Funding from the Carbon to Sea Initiative has fueled the development of practical solutions, but much more support is needed to rapidly vet and scale this technology,” says de Lannoy.
Policy and regulation surrounding oceans is another, sensitive hurdle, especially when it comes to adopting novel, innovative solutions. But de Lannoy and Abdelkader feel that the risk of inaction greatly outweighs the risk of adoption of BMED technology.
They enlisted a group of chemical engineering undergraduate students including Allison Suichies, Huilin Liu, Jiayue Zhu, Lucas Ning and Youssef Hassanin, to complete a technical analysis as part of the students’ final capstone project – a requirement for graduation.

The final-year students worked to determine the viability of building a plant to support BMED in Ontario, Nova Scotia and British Columbia today, and other considerations like what to do with the dialysis byproduct, minimizing cost drivers and energy requirements.
“This work has the potential to stimulate natural ocean carbon capture and lessen the effects of ocean acidification through a single integrated framework,” says Suichies, who is employed as a research assistant in the de Lannoy Lab. “I am so grateful to Dr. de Lannoy and Dr. Abdelkader for their sharing of knowledge and for this opportunity to continue learning and continue contributing to a more sustainable future.
The impacts of climate change on our oceans demands our attention. With proven, tested, and safe technologies we hope to stimulate more conversation around the need for CO2 removal in addressing climate change.